资源目录
压缩包内文档预览:(预览前20页/共44页)
编号:18009933
类型:共享资源
大小:5.93MB
格式:RAR
上传时间:2019-04-23
上传人:闰***
认证信息
个人认证
冯**(实名认证)
河南
IP属地:河南
60
积分
- 关 键 词:
-
Proe三维
花生剥壳去壳机设计
三维PROE
花生剥壳机花生去壳机
花生去壳机设计
- 资源描述:
-

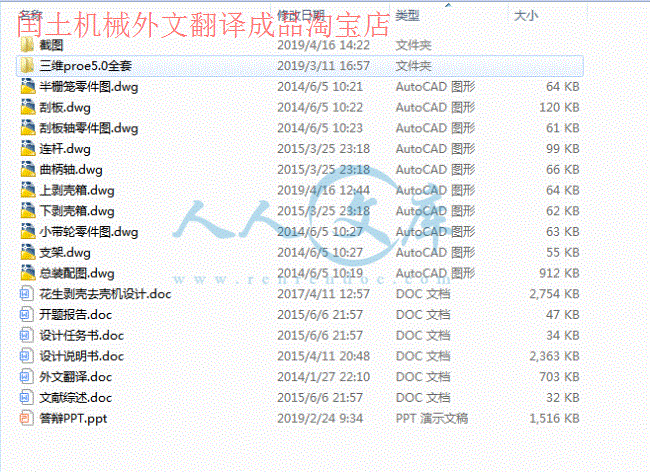





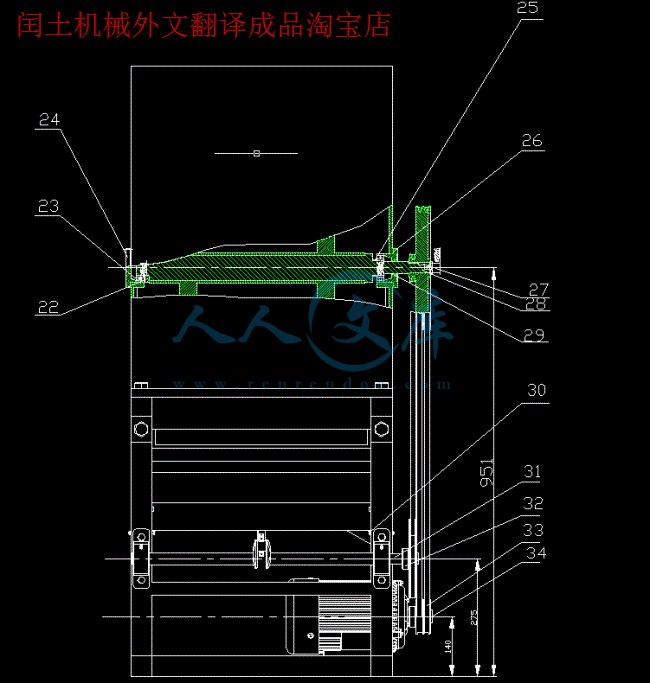



- 内容简介:
-
附录 英文翻译资料中文翻译正电原子在电离过程中碰撞的理论摘要 我们回顾过去和现在正子原子在电离过程中碰撞理论的发展。 从最终状态下合并所有相互作用,在一个同等立足处和保留少量碰撞动力学的一个确切的物体分析开始, 我们进行或重或轻不同的比较, 并且从它们影响电离横剖面的角度进行分析。 终于, 我们发现了理论碰撞过程中的连续统一体, 中心点和其它运动学机制 。主题词: 电离; 碰撞动力学; 驱散; 电子光谱; 反物质; 正电子冲击; 中心点电子; 导轨式电子1. 介绍 正电原子的简单电离碰撞由一个细小的结构微粒冲击, “三体问题”是很多年未解决的一个物理问题。 1609 年到1687 年“二体”问题由约翰尼.开普勒和由艾萨克牛顿共同解决了。三体问题比二体问题更加复杂难懂, 除了一些特殊的现象,它不能被简单的分析解决。 1765年, 勒翰得. 依鲁尔发现了原始在线的三大量和依然排列的一种几何 解答。不少年后, 拉格朗日发现了五平衡点的存在, 今后大家都称为拉格朗日点。 对三体驱散问题的解答,最早的是三百年前天文学家和数学家用数学工具和相似比的原理解答出来的。例如, 在大量的中心参考系统下, 我们在1836 年描述三体问题由任何空间座标都可能的原因已经由杰库比介绍。所有这些对由线形点标准变革关系, 如所描述 1 。在动量空间, 系统由伴生的描述(千吨), (千焦) 和(千牛) 。 交换对实验室参考框架, 大量电子最后的动量m, 许多MT (反冲) 目标片段和大量MP 子弹头可能被写根据杰克比冲动Kj 通过伽利略变换 1 得出数十年, 电离过程的理论描述承担了三体动力学在最终状态下的简单表示, 根据事实表明 (1)对于离子和原子碰撞, 一个微粒(电子) 比其它二两个原子要轻。 (2)对于电子和正子原子碰撞, 一个微粒(目标中坚力量) 比其它两个原子要重的多。 例如, 根据众所周知的中心论据, 离子和原子电离碰撞的理论描述的决大多数使用冲击参数来设置, 那里子弹头跟随一条未受干扰的直线弹道在碰撞过程过程中, 并且目标中坚力量依然是休息 2 。 它是确切, 假设, 子弹头随后而来一条直线弹道没有道理在电子或正子原子碰撞的理论描述。 但是, 它通常假设, 目标中坚力量依然是不动。 问题的这些简单化被介绍了在18 世纪。 unsolvable t三体问题被简化了, 对所谓的有限的三体问题, 那里一个微粒被承担有一许多足够小不影响其它二个微粒的行动。 虽则介绍作为手段提供近似解答对系统譬如太阳行星彗星在古典技工范围内, 它广泛被应用在原子物理在所谓的冲击参量略计对离子原子电离碰撞。 三体问题的其它简单化广泛被使用在19 世纪假设, 一个微粒比其它二巨型的并且依然是在大量的中心镇定自若由其它二。 这略计广泛被应用在电子或正子原子电离碰撞。2. 多个有差别的横剖面 一个三体连续流最后状态的一个运动学上完全描述在任一原子碰撞会要求, 原则上, 九可变物知识, 譬如动量的组分联系了对每个三个微粒在最终状态。 但是, 动量和能源节约的情况减少这个数字 到五。 此外, 每当最初的目标不准备在任何优先方向, 多个有差别的横剖面必须是相称由三体系统的自转在子弹头的行动的最初的方向附近。 因而, 搁置一边三个片段的内部结构在最终状态, 只四 丧失九可变物是必要完全地描述驱散过程。 所以, 电离过程的一个完全描述特性也许被获得以一个四倍有差别的横剖面:有许多可能的套四可变物使用。 为,事例, 我们能选择了电子的方位角角度和其它二个微粒的当中一个, 相对角度在行动之间飞机, 并且一个微粒能量。这样选择是任意的, 但完成在感觉, 其他套可变物可能与这一个有关。 独立可变物一个相似的选择是标准的为原子电离的描述由电子冲击, 理论上和实验性地 3,4 。 非常一般四倍有差别的横剖面的图片不是可行的。 因而, 它通常是必要减少可变物的数量在横剖面。 这可能由修理达到一两他们在某些特殊价值或情况。 例如, 我们也许任意地制约自己描述coplanar (i.e. =0) 或a collinear motion (i.e. =0 and 1=2), 以便使问题的依赖性降低到三或二独立可变物, 各自地。 另一选择将集成四倍有差别的横剖面在一个或更多可变物。前广泛被应用学习电子碰撞, 当后者是主要工具描绘离子原子和正子原子电离碰撞。 特别重要对唯一微粒分光学的用途, 那里动量的微粒的当中一个被测量。 3. 单个微粒的动量分布 动量发行为散发的电子和正子礼物几个结构。 首先, 我们能观察门限在高电子或正子速度因为有一个极限在任一个微粒可能吸收从系统的动能。 第二个结构是土坎被设置沿圈子。 它对应于正子的二进制碰撞与散发的电子, 用目标中坚力量充当实际角色。 终于, 有尖顶和anticusp 在零速度在电子和正子动量分布, 各自地。 第一个对应于电子的励磁于目标的一个低能源连续流状态。秒钟是取尽由于正子的捕获的不可能的事由目标中坚力量。 这些动量发行允许我们学习电离碰撞的主要特征。 但是, 我们必须记住, 分析只微粒的当中一个在最后状态的任一个实验性技术可能只提供部份洞察入电离过程。 四倍有差别的横剖面也许显示由综合化洗涤在这实验的碰撞物产。4. 理论模型我们想要讨论在这通信的主要问题是如果有一些重要碰撞物产在正子原子碰撞, 那不是可测的,总共, 单或双有差别的电离横剖面, 并且那因为未被发现。 为了了解这些结构的起源, 我们对应的横剖面与那些比较被获得在离子原子碰撞。 履行这个宗旨它是必要的有一种充分的量子机械治疗能同时应付电离碰撞由重和轻的子弹头的冲击是因此相等地可适用的- 例如- 对离子原子或正子原子碰撞。 一种理论与这特征将允许我们学习倍数任一个指定的特点的变动有差别的横断面当许多联系在片段之中变化。 特别是, 它会允许我们学习变异当改变在二之间制约了运动学情况。 第二重要点将对待所有互作用在最终状态在一个同等立足处。 如同我们解释了, 在离子原子碰撞, internuclear 互作用不充当实际在散发的电子的动量发行的角色和因此未被考虑在对应的演算。 在这工作, 这假定被避免了。 横剖面利益在这范围内是转折矩阵可能供选择地被写在岗位或预先的形式那里扰动潜力被定义为出生类型初始状态哪些包括子弹头的自由行动和最初的一定的状态Ui 目标, 并且扰动潜力vi 简单地是正子电子和正子中坚力量互作用的总和。 转折矩阵也许然后被分解入二个期限依靠是否正子首先与目标中坚力量或电子相处融洽。为了是一致的与动力学的我们充分的治疗, 它是必要描述最终状态Wf 通过考虑所有互作用在同样立足处的wavefunction 。 因而, 我们采取一个被关联的C3 波浪作那包括畸变Dj 为三活跃互作用。 在连续流波浪作用这个选择的最后渠道扰动潜力是 5 在纯净的库仑潜力情况下, 畸变被给关于这个模型由佳瑞波帝和马瑞吉拉 6 提议为离子原子碰撞, 并且由Brauner 和布里格斯六年后为正子原子和电子碰撞 7 。 但是, 在所有这些箱子问题的动力学被简化了, 依照被谈论在早先部分, 根据大非对称在介入的片段的大量之间。 另外, Garibotti 和Miraglia 忽略了互作用潜力的矩阵元素在接踵而来的子弹头和目标离子之间, 并且做锐化的略计评估转折矩阵元素。 这进一步略计被取消了在纸由Berakdar 等。 (1992), 虽然他们保留许多制约在他们的离子冲击电离分析。 5. 电子捕获对连续流尖顶 让我们回顾一些结果在立体几何。 我们选择作为二个独立参量散发的电子动量组分, 平行和垂线对正子子弹头的行动的最初的方向。 子弹头的能量是1 keV 。图2, 我们观察三个不同结构: 二个极小值和土坎。图2 土坎的起源很好被了解。 它对应于电子捕获于连续流(ECC) 尖顶被发现在离子原子碰撞三十年前由Crooks 和Rudd 8 。 他们测量了电子能量光谱在向前方向和确切地观察了尖顶形状峰顶在子弹头的速度。 第一理论解释 9 表示, 它分流以与1 相似的方式k 。 这个尖顶结构是很多实验性和理论研究焦点。 因为ECC 尖顶是一个推测横跨捕获电离极限入高度激动的一定的状态, 这个同样作用必须是存在在正子原子碰撞。 实际上, 这样作用的观察联系了假定物体的形成, 当被预言的二十年前由布朗勒和布里格斯, 依然是一个有争议的问题。 这争执的原因是那, 与离子对比盒, 正子外出的速度与那不是相似冲击, 但主要传播在角度和巨大。 因而没有特殊速度在哪里寻找尖顶。 并且这一定是如此。 如果我们评估双重有差别的横剖面, 我们看见, 尖顶清楚地是可看见的在离子原子碰撞, 但非常温和和被传播的肩膀在正子原子碰撞。 因而, 观察这结构它是必要增加横剖面的维度。 例如由考虑四倍有差别的横剖面的零的程度裁减在collinear 几何。Kover 和Laricchia 测量了在1998 dr/dEedXkdXK 横剖面在一个collinear 情况在零的程度, 为H2 的电离分子由100 keV 正子冲击 10 。 结构依照为冲击对重的离子被观察那么尖锐不被定义由于占实验性窗口在正子的卷积 并且电子侦查。 从目标反冲不充当在这个实验性情况的重大角色, 当前一般理论给结果相似与那些由Berakdar 11 获得, 并且两个跟随严密实验性价值。 这同样实验由Sarkadi 和工友执行了在氩电离由75 keV 氢核冲击。 他们第一次测量了四倍有差别的电离横剖面在collinear 几何为离子原子碰撞, 并且发现ECC 尖顶和在正子冲击在大角度。 在这种情况下, 我们必须保留动力学的一个完全帐户为了再生产实验性结果 12 。6. 托马斯机制 现在让我们走回到H2 的电离由1 keV 正子冲击。 一个结构在45 可能被观察, 1993 年哪些象由于被预言了和被解释了由Brauner 和布里格斯二个等效双重碰撞机制干涉。 每个这些过程包括正子电子二进制碰撞, 被偏折跟随被90 轻的微粒的当中一个被重的中坚力量。 这个机制由托马斯 13 提议作为扼要负责任电子捕获由快速的重的离子。 在这种情况下, 从电子和正子大量是相等的, 这两个过程干涉在45 。 如果我们降低能量从1000 年eV 到100 eV, 这个结构在45 消失, 与想法是一致的结果托马斯机制是一个高能作用。 但有其它结构, 在大约22.5。我们在下个部分将考虑这个结构。7. 备鞍点机制 结构的起源在大约22.5 一定更难辨认。 对我们的最佳的知识, 它以前未被预言在正子原子碰撞, 即使机制负责任它的起源几乎已经提议在离子原子碰撞二十年之内以前。 想法是, 电子能从离子原子碰撞涌现由在在子弹头和残余的目标离子潜力的备鞍点。 1772 年这个机制清楚地与平衡点的当中一个有关由拉格朗日发现, 或对机制由Wannier 提议为低能源电子放射。 在 离子原子碰撞案件, 查寻这个机制的理论和实验性证据是阴暗由生动的争论 14-18 。在正子原子碰撞情况下, 为电子被困住在正子和残余离子潜力的马鞍, 电子和正子必须首先执行二进制碰撞以便最终获得正确的速度那里ei 是目标的结合能在初始状态。 能量和动量保护原则的应用表示, 正子偏离在角度 终于, 为电子涌现在方向和正子一样, 它必须遭受随后碰撞以残余中坚力量在a 托马斯象过程。 在这第二碰撞, 电子由90 和残余目标离子反冲偏转在形成大约135 角度与电子和正子的方向。 这个机制被描述在图4. 因而, 检查备鞍点的提案是正确的, 我们看是否我们的演算显示与备鞍点电子生产的这个描述是一致的结构。 图 3 图 4 极小值被观察在无效性QDCS 。 图3 和图4 精确地设置早先条件在任何能量和角度三个微粒符合的那些点。 我们做了其它测试在备鞍点机制的有效性和无效性。 图5 表示, 结构完全出现从tp 期限。 这个结果与提出的机制是一致的, 那里备鞍点结构出现从第一正子电子碰撞之后, 正子和电子被中坚力量驱散。 图 58. 结论 总结结果提出了在这通信, 我们由正子的冲击调查了分子氢的电离。 被获得的四倍有差别的横断面为电子和正子涌现在同样方向显示三个统治结构。 你是知名的电子捕获对连续流峰顶。 另外一个是托马斯机制。 终于, 有被解释对象由于所谓的备鞍点 电离机制的极小值。 虽然主要结论研究的非常充分但也有一些不足。横剖面也许会被很多巨大的困难所阻碍, 但值得高兴的是, 我们一直没有错过对问题许多不同的全方位的观察, 唯一的遗憾就是对总横剖面的研究。英文原文Theory of ionization processes in positronatom collisionsAbstract We review past and present theoretical developments in the description of ionization processes in positronatom collisions. Starting from an analysis that incorporates all the interactions in the final state on an equal footing and keeps an exact account of the few-body kinematics, we perform a critical comparison of different approximations, and how they affect the evaluation of the ionization cross section. Finally, we describe the appearance of fingerprints of capture to the continuum, saddle-point and other kinematical mechanisms. Keywords: Ionization; Collision dynamics; Scattering; Electron spectra; Antimatter; Positron impact; Saddle-point electrons; Wannier; CDW PACS classification codes: 34.10.+x; 34.50.Fa 1. Introduction The simple ionization collision of a hydrogenic atom by the impact of a structureless particle, the “three-body problem”, is one of the oldest unsolved problems in physics. The two-body problem was analyzed by Johannes Kepler in 1609 and solved by Isaac Newton in 1687. The three-body problem, on the other hand, is much more complicated and cannot be solved analytically, except in some particular cases. In 1765, for instance, Leonhard Euler discovered a “collinear” solution in which three masses start in a line and remain lined-up. Some years later, Lagrange discovered the existence of five equilibrium points, known as the Lagrange points. Even the most recent quests for solutions of the three-body scattering problem use similar mathematical tools and follow similar paths than those travelled by astronomers and mathematicians in the past three centuries. For instance, in the center-of-mass reference system, we describe the three-body problem by any of the three possible sets of the spatial coordinates already introduced by Jacobi in 1836. All these pairs are related by lineal point canonical transformations, as described in 1. In momentum space, the system is described by the associated pairs (kT,KT), (kP,KP) and (kN,KN). Switching to the Laboratory reference frame, the final momenta of the electron of mass m, the (recoil) target fragment of mass MT and the projectile of mass MP can be written in terms of the Jacobi impulses Kj by means of Galilean transformations 1For decades, the theoretical description of ionization processes has assumed simplifications of the three-body kinematics in the final state, based on the fact that in an ionatom collision, one particle (the electron) is much lighter than the other two, in an electronatom or positronatom collision, one particle (the target nucleus) is much heavier than the other two.For instance, based on what is known as Wicks argument, the overwhelming majority of the theoretical descriptions of ionatom ionization collisions uses an impact-parameter approximation, where the projectile follows an undisturbed straight line trajectory throughout the collision process, and the target nucleus remains at rest 2. It is clear that to assume that the projectile follows a straight line trajectory makes no sense in the theoretical description of electron or positronatom collisions. However, it is usually assumed that the target nucleus remains motionless. These simplifications of the problem were introduced in the eighteenth century. The unsolvable three-body problem was simplified, to the so-called restricted three-body problem, where one particle is assumed to have a mass small enough not to influence the motion of the other two particles. Though introduced as a means to provide approximate solutions to systems such as Sunplanetcomet within a Classical Mechanics framework, it has been widely used in atomic physics in the so-called impact-parameter approximation to ionatom ionization collisions. Another simplification of the three-body problem widely employed in the nineteenth century assumes that one of the particles is much more massive than the other two and remains in the center of mass unperturbed by the other two. This approximation has been widely used in electronatom or positronatom ionization collisions. 2. The multiple differential cross section A kinematically complete description of a three-body continuum final-state in any atomic collision would require, in principle, the knowledge of nine variables, such as the components of the momenta associated to each of the three particles in the final state. However, the condition of momentum and energy conservation reduces this number to five. Furthermore, whenever the initial targets are not prepared in any preferential direction, the multiple differential cross section has to be symmetric by a rotation of the three-body system around the initial direction of motion of the projectile. Thus, leaving aside the internal structure of the three fragments in the final state, only four out of nine variables are necessary to completely describe the scattering process. Therefore, a complete characterization of the ionization process may be obtained with a quadruple differential cross section:There are many possible sets of four variables to use. For, instance, we can chose azimuthal angles of the electron and of one of the other two particles, the relative angle between the planes of motion, and the energy of one particle.Such a choice is arbitrary, but complete in the sense that any other set of variables can be related to this one. A similar choice of independent variables has been standard for the description of atomic ionization by electron impact, both theoretically and experimentally 3 and 4. A picture of the very general quadruple differential cross section is not feasible. Thus, it is usually necessary to reduce the number of variables in the cross section. This can be achieved by fixing one or two of them at certain particular values or conditions. For instance, we might arbitrarily restrict ourselves to describe a coplanar (i.e. =0) or a collinear motion (i.e. =0 and 1=2), so as to reduce the dependence of the problem to three or two independent variables, respectively. The other option is to integrate the quadruple differential cross section over one or more variables.The former has been widely used to study electronatom collisions, while the latter has been the main tool to characterize ionatom and positronatom ionization collisions. Particularly important has been the use of single particle spectroscopy, where the momentum of one of the particles is measured. 3. Single particle momentum distributions In ionization by positron impact it is feasible to study the momentum distribution of any of the involved fragments. As is shown in Fig. 1, the momentum distributions for the emitted electron and the positron present several structures. First, we can observe a threshold at high electron or positron velocities because there is a limit in the kinetic energy that any particle can absorb from the system. The second structure is a ridge set along a circle. It corresponds to a binary collision of the positron with the emitted electron, with the target nucleus playing practically no role. Finally, there is a cusp and an anticusp at zero velocity in the electron and positron momentum distributions, respectively. The first one corresponds to the excitation of the electron to a low-energy continuum state of the target. The second is a depletion due to the impossibility of capture of the positron by the target nucleus. These momentum distributions allow us to study the main characteristics of ionization collisions. However, we have to keep in mind that any experimental technique that analyzes only one of the particles in the final-state can only provide a partial insight into the ionization processes. The quadruple differential cross sections might display collision properties that are washed out by integration in this kind of experiments. Fig. 1.Electron and positron momentum distributions for the ionization of helium by impact of positrons with incident velocity v=12a.u. 4. Theoretical model The main question that we want to address in this communication is if there are some important collision properties in positronatom collisions, that are not observable in total, single or double differential ionization cross sections, and that therefore have not yet been discovered. In order to understand the origin of these structures, we compare the corresponding cross sections with those obtained in ionatom collisions. To fulfill this objective it is necessary to have a full quantum-mechanical treatment able to deal simultaneously with ionization collisions by impact of both heavy and light projectiles that is therefore equally applicable for instance to ionatom or positronatom collisions. A theory with this characteristics will allow us to study the changes of any given feature of multiple-differential cross-sections when the mass relations among the fragments vary. In particular, it would allow us to study the variation when changing between the two restricted kinematical situations. The second important point is to treat all the interactions in the final state on an equal footing. As we have just explained, in ionatom collisions, the internuclear interaction plays practically no role in the momentum distribution of the emitted electron and has therefore not been considered in the corresponding calculation. In this work, this kind of assumption has been avoided. The cross section of interest within this framework isThe transition matrix can be alternatively written in post or prior forms aswhere the perturbation potentials are defined by (HE)i=Vi i and (HE)f=Vff. For the Born-type initial statewhich includes the free motion of the projectile and the initial bound state i of the target, and the perturbation potential Vi is simply the sum of the positronelectron and positronnucleus interactions. The transition matrix may then be decomposed into two termsdepending on whether the positron interacts first with the target nucleus or the electron. In order to be consistent with our full treatment of the kinematics, it is necessary to describe the final state by means of a wavefunction that considers all the interactions on the same footing. Thus, we resort to a correlated C3 wave functionthat includes distortions for the three active interactions. The final-channel perturbation potential for this choice of continuum wave function is 5(1)In the case of pure coulomb potentials, the distortions are given bywith j=mjZj/kj. This model was proposed by Garibotti and Miraglia 6 for ionatom collisions, and by Brauner and Briggs six years later for positronatom and electronatom collisions 7. However, in all these cases the kinematics of the problem was simplified, as discussed in the previous section, on the basis of the large asymmetry between the masses of the fragments involved. In addition, Garibotti and Miraglia neglected the matrix element of the interaction potential between the incoming projectile and the target ion, and made a peaking approximation to evaluate the transition matrix element. This further approximation was removed in a paper by Berakdar et al. (1992), although they kept the mass restrictions in their ion-impact ionization analysis. 5. The electron capture to the continuum cusp Let us review some results in a collinear geometry. We choose as the two independent parameters the emitted electron momentum components, parallel and perpendicular to the initial direction of motion of the positron projectile. The energy of the projectile is 1keV. In Fig. 2, we observe three different structures: two minima and a ridge. Fig. 2.QDCS for ionization of H2 by impact of 1keV positrons for emission of electrons in the direction of the projectile deflection.The origin of the ridge is very well understood. It corresponds to the electron capture to the continuum (ECC) cusp discovered in ionatom collisions three decades ago by Crooks and Rudd 8. They measured the electron energy spectra in the forward direction and observed a cusp-shape peak at exactly the projectiles velocity. The first theoretical explanation 9 showed that it diverges in the same way as 1/k. This cusp structure was the focus of a large amount of experimental and theoretical research. Since the ECC cusp is an extrapolation across the ionization limit of capture into highly excited bound states, this same effect has to be present in positronatom collisions. In fact, the observation of such an effect associated with positronium formation, while predicted two decades ago by Brauner and Briggs, remained a controversial issue. The reason for this dispute was that, in contrast to the case of ions, the positron outgoing velocity is not similar to that of impact, but is largely spread in angle and magnitude. Thus there is no particular velocity where to look for the cusp. And this is certainly so. If we evaluate the double differential cross section, we see that the cusp is clearly visible in ionatom collisions, but just a very mild and spread shoulder in positronatom collisions. Thus, to observe this structure it is necessary to increase the dimension of the cross section. For instance by considering a zero degree cut of the quadruple differential cross section in collinear geometry. Kover and Laricchia measured in 1998 the d/dEedkdK cross section in a collinear condition at zero degree, for the ionization of H2 molecules by 100keV positron impact 10. The structure is not so sharply defined as for impact observed for heavy ions because of the convolution that accounts for the experimental window in the positron and electron detection. Since the target recoil plays no significant role in this experimental situation, the present general theory gives results similar to those obtained by Berakdar 11, and both closely follow the experimental values. The same kind of experiment was performed by Sarkadi and coworkers in Argon ionization by 75keV proton impact. They measured the quadruple differential ionization cross section in a collinear geometry for ionatom collisions for the first time, and found the ECC cusp as in positron impact at large angles. In this case, we have to keep a complete account of the kinematics in order to reproduce the experimental results 12. 6. Thomas mechanism Let us now go back to the ionization of H2 by 1keV positron impact. A structure at 45 can be observed, which was predicted and explained in 1993 by Brauner and Briggs as due to the interference of two equivalent double-collision mechanisms. Each of these processes consists of a positronelectron binary collision, followed by the deflection by 90 of one of the light particles by the heavy nucleus. This mechanism was proposed by Thomas 13 as the main responsible of electron capture by fast heavy ions. In this case, since the electron and positron masses are equal, these two processes interfere at 45. If we lower the energy from 1000eV to 100eV, this structure at 45 disappears, a result that is consistent with the idea that the Thomas mechanism is a high energy effect. But there is another structure, at about 22.5, that persists. We will consider this structure in the next section. 7. Saddle-point mechanism The origin of the structure at about 22.5 is certainly more difficult to identify. To our best knowledge, it has not been predicted before in positronatom collisions, even though the mechanism responsible of its origin was already been proposed in ionatom collisions almost two decades before. The idea was that an electron could emerge from an ionatom collision by lying in the saddle-point of the projectile and the residual target-ion potentials. This mechanism is clearly related to one of the equilibrium points discovered by Lagrange in 1772, or to the mechanism proposed by Wannier for low-energy electron emission. In the case of ionatom collisions, the search for theoretical and experimental evidence of this mechanism was overcast by vivid controversy 14, 15, 16, 17 and 18. In the case of positronatom collisions, for the electrons to be trapped in the saddle of the positron and residual-ion potentials, the electron and the positron must first perform a binary collision s
- 温馨提示:
1: 本站所有资源如无特殊说明,都需要本地电脑安装OFFICE2007和PDF阅读器。图纸软件为CAD,CAXA,PROE,UG,SolidWorks等.压缩文件请下载最新的WinRAR软件解压。
2: 本站的文档不包含任何第三方提供的附件图纸等,如果需要附件,请联系上传者。文件的所有权益归上传用户所有。
3.本站RAR压缩包中若带图纸,网页内容里面会有图纸预览,若没有图纸预览就没有图纸。
4. 未经权益所有人同意不得将文件中的内容挪作商业或盈利用途。
5. 人人文库网仅提供信息存储空间,仅对用户上传内容的表现方式做保护处理,对用户上传分享的文档内容本身不做任何修改或编辑,并不能对任何下载内容负责。
6. 下载文件中如有侵权或不适当内容,请与我们联系,我们立即纠正。
7. 本站不保证下载资源的准确性、安全性和完整性, 同时也不承担用户因使用这些下载资源对自己和他人造成任何形式的伤害或损失。

人人文库网所有资源均是用户自行上传分享,仅供网友学习交流,未经上传用户书面授权,请勿作他用。