多用角架搁板的注塑模具设计及其仿真加工设计
50页 21000字数+说明书+外文翻译+9张CAD图纸【详情如下】
凸模.dwg
凹模.dwg
动模固定板.dwg
塑件.dwg
外文翻译--对通过塑料注射成型零件的选择性激光融化生产得到的功能梯度材料插入棒的评估 中文版.doc
外文翻译--对通过塑料注射成型零件的选择性激光融化生产得到的功能梯度材料插入棒的评估 英文版.pdf
多用角架搁板.doc
多用角架搁板的注塑模具设计及其仿真加工设计.doc
定模座板.dwg
导套.dwg
拉料杆A4.dwg
浇口套.dwg
装配图.dwg
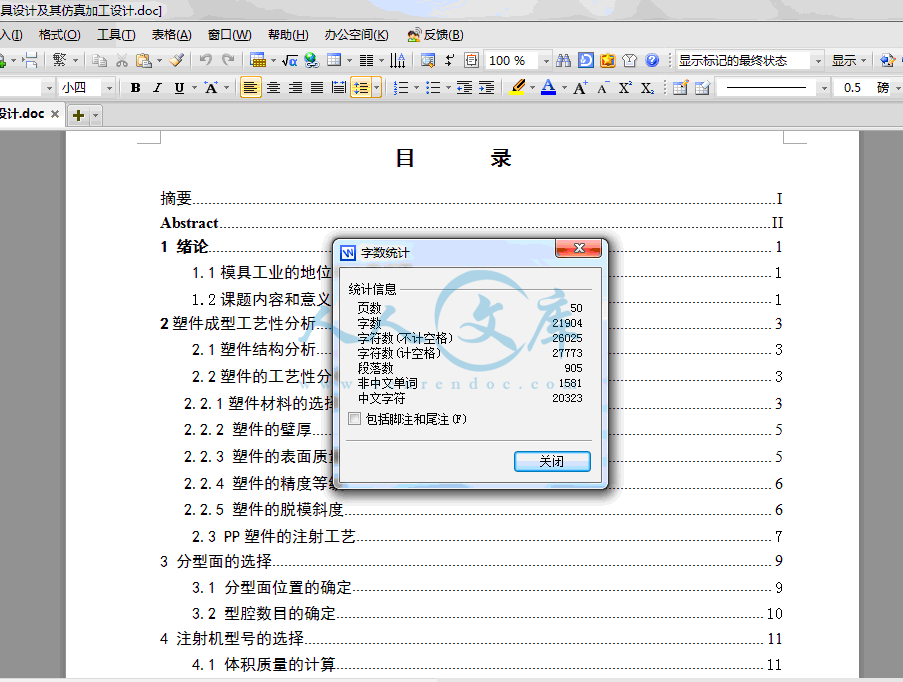
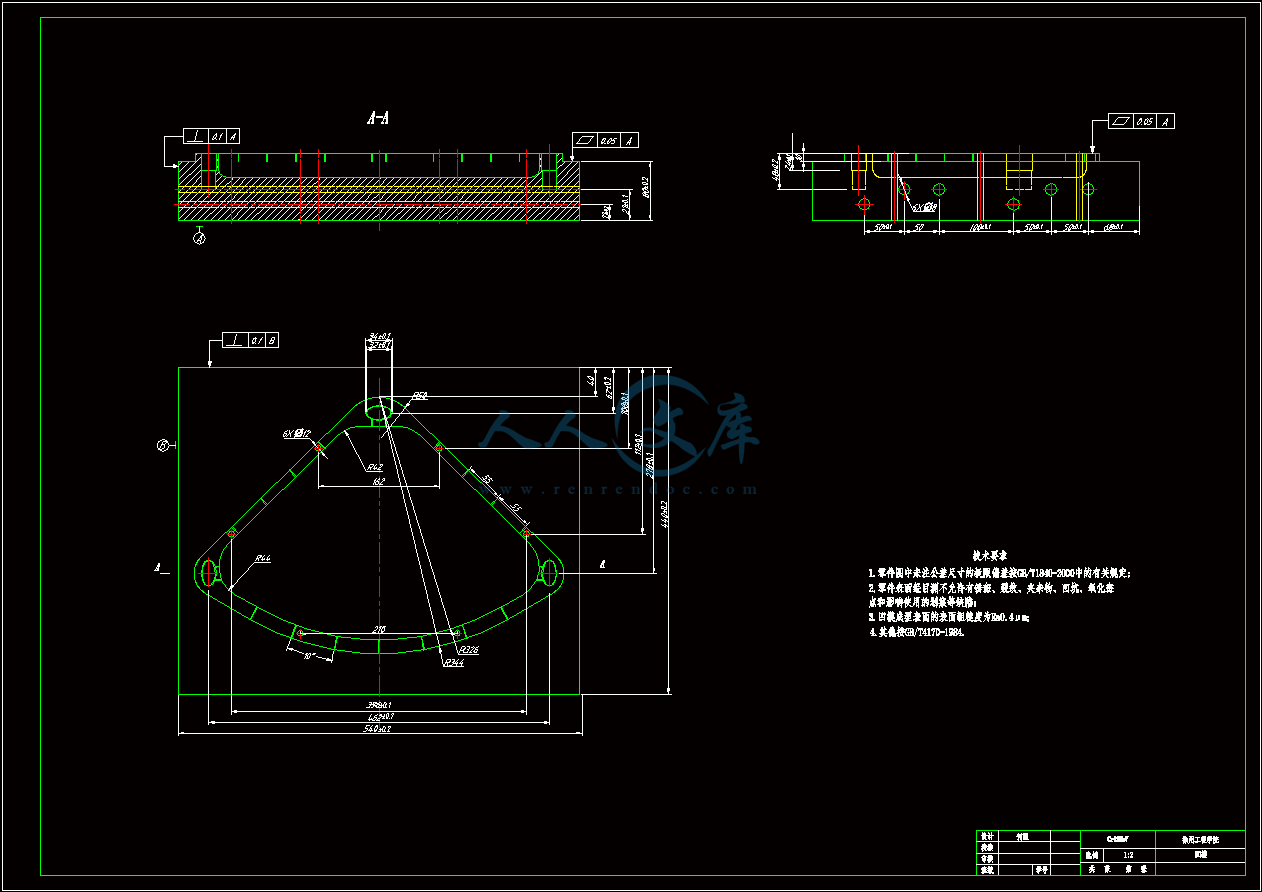

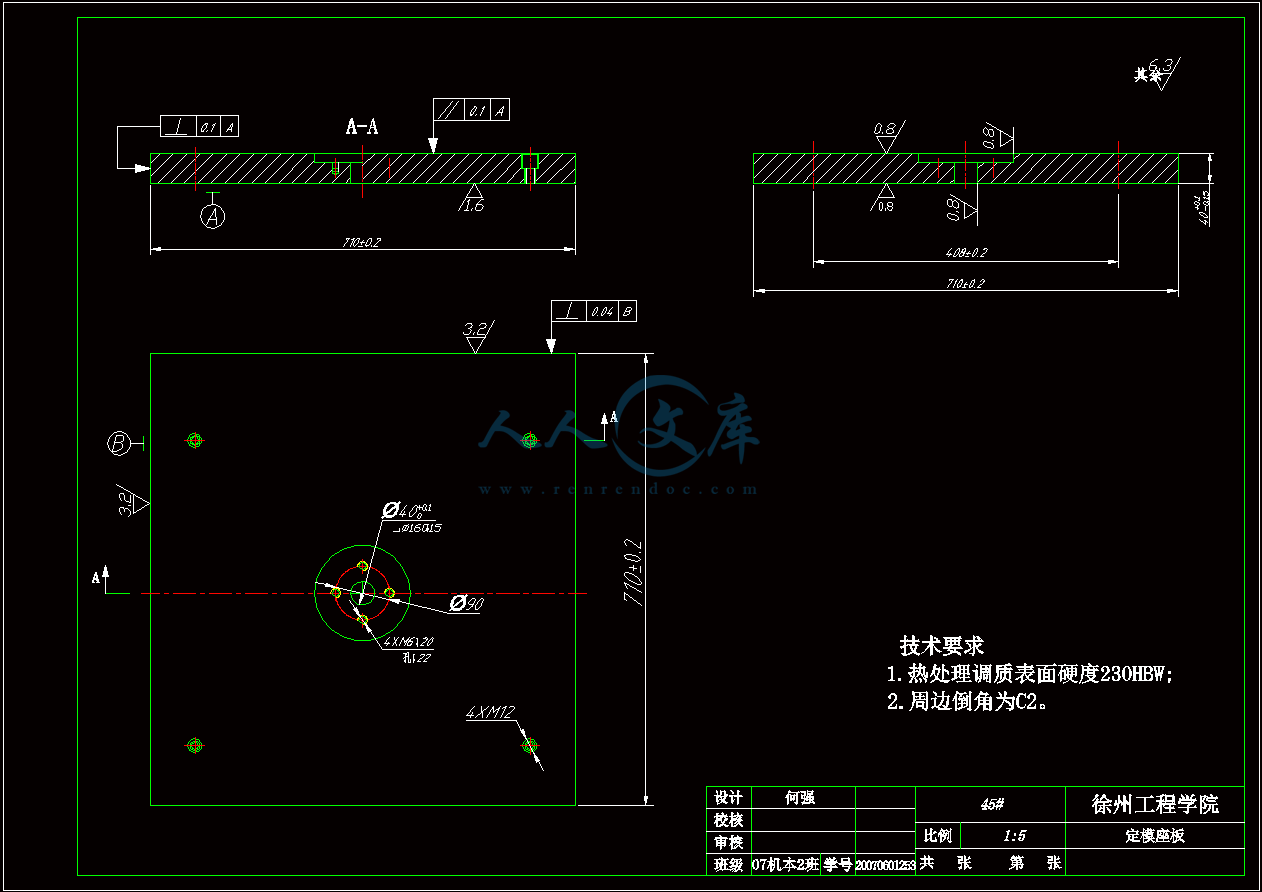

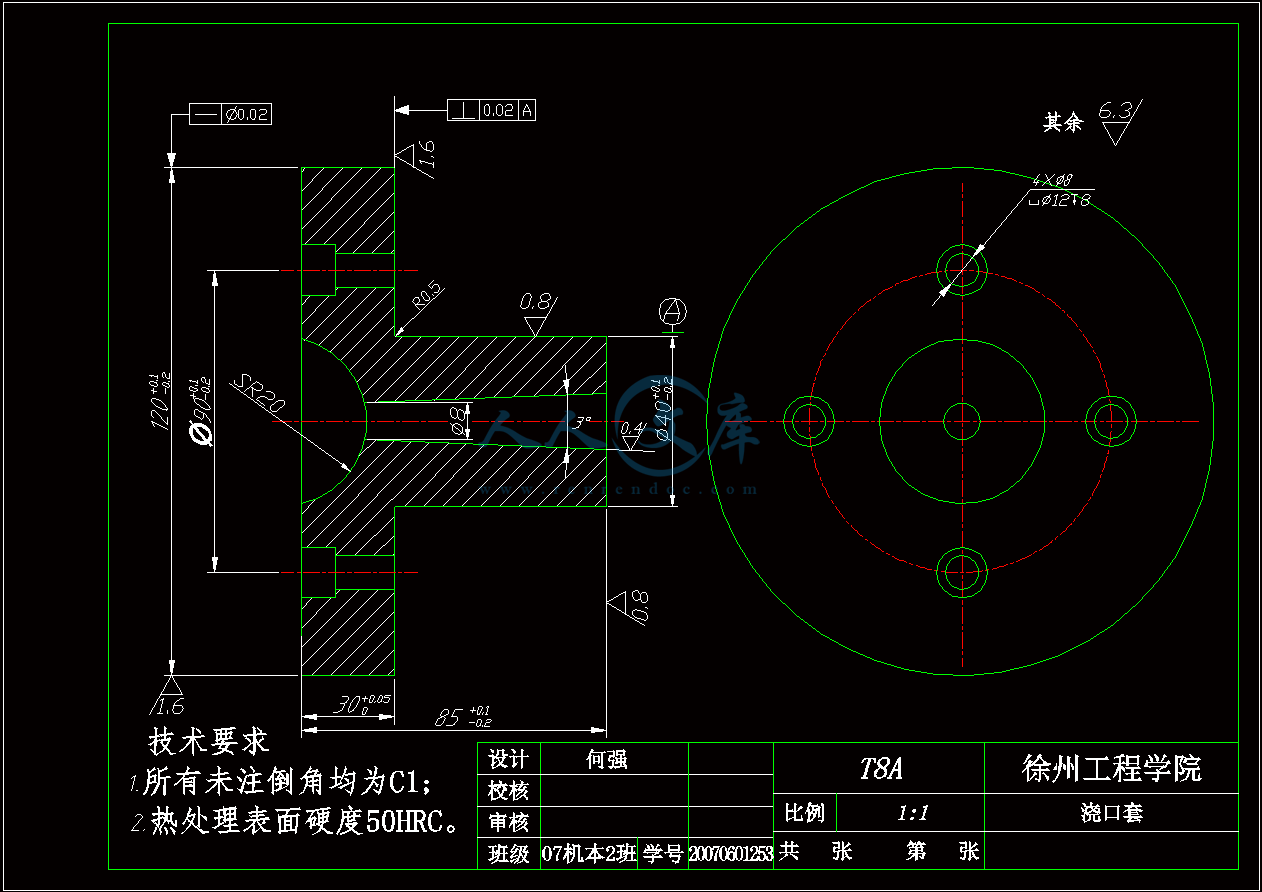


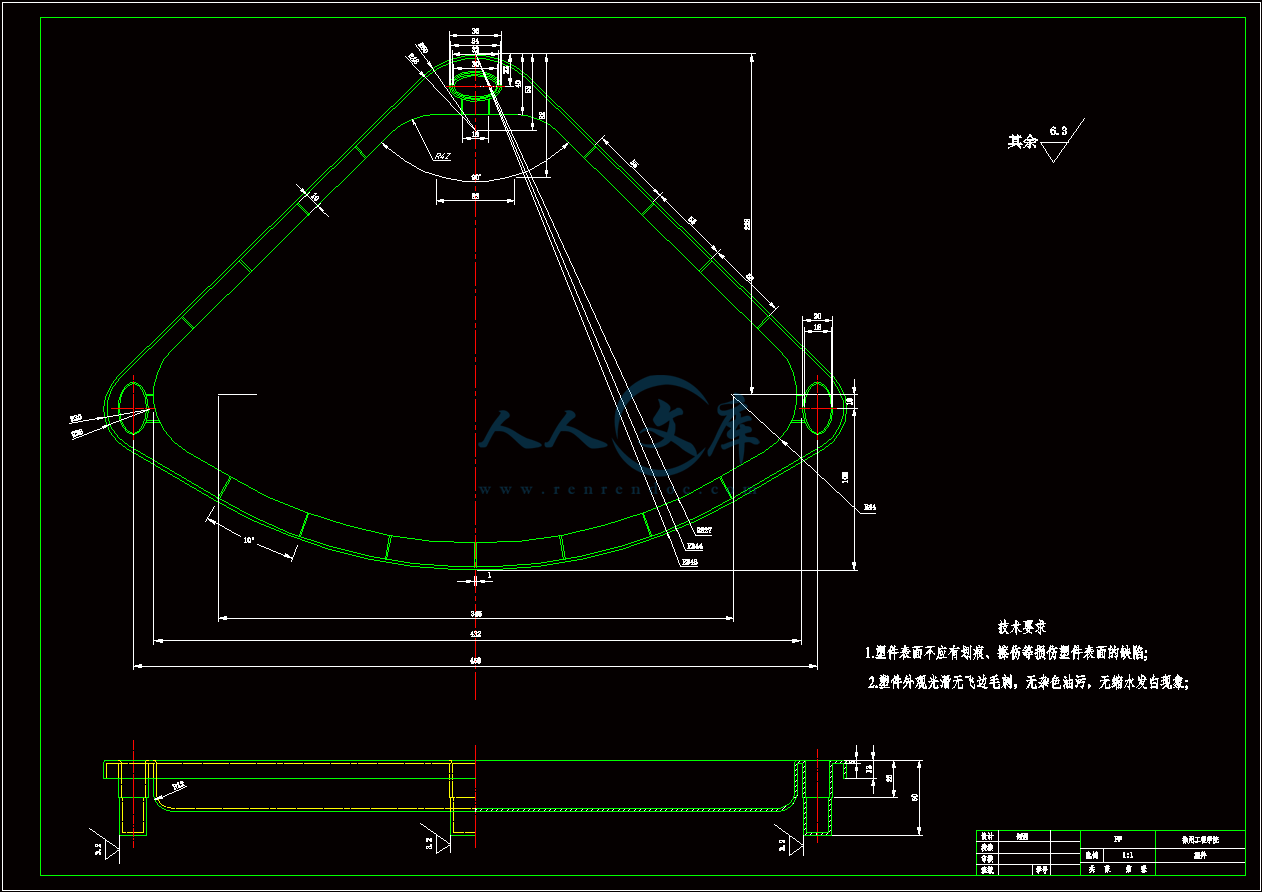
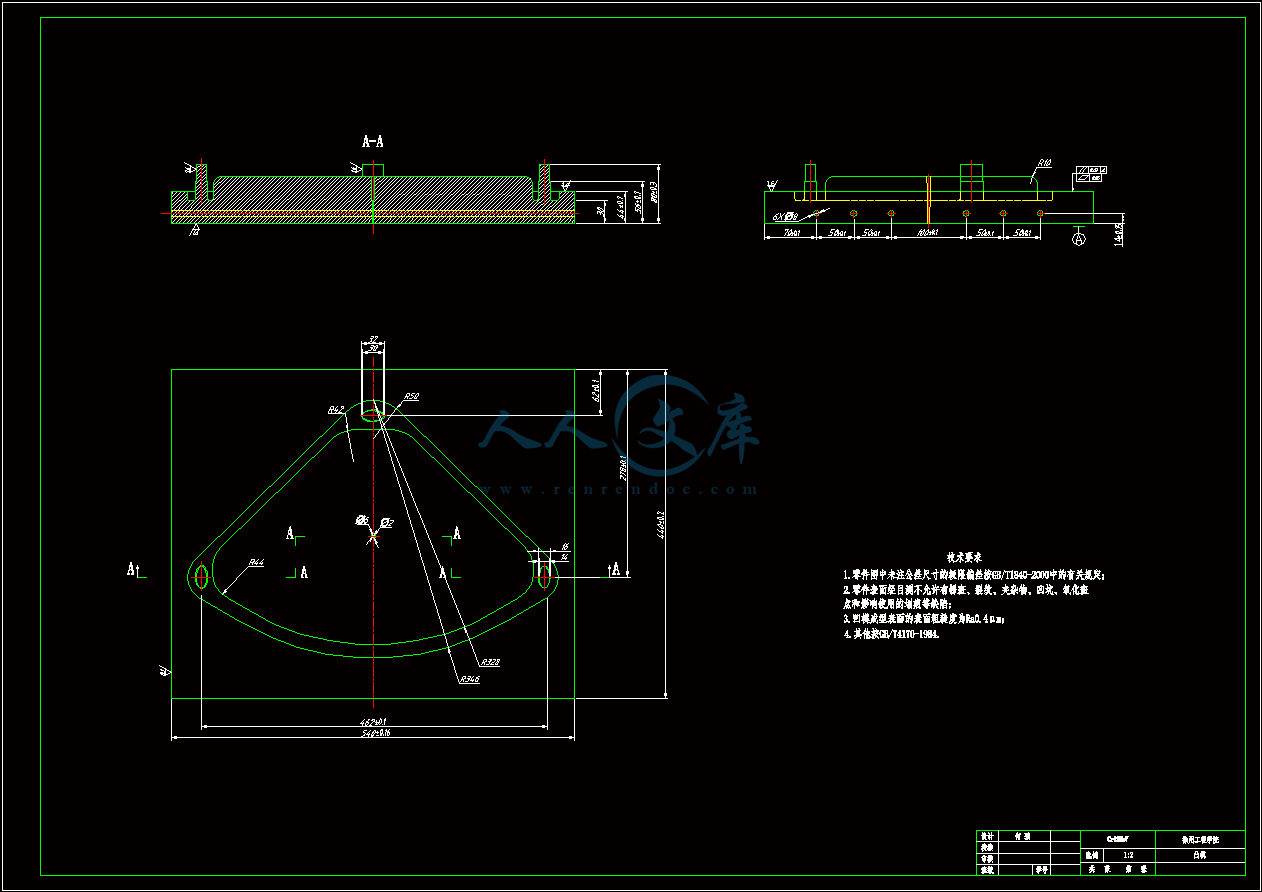
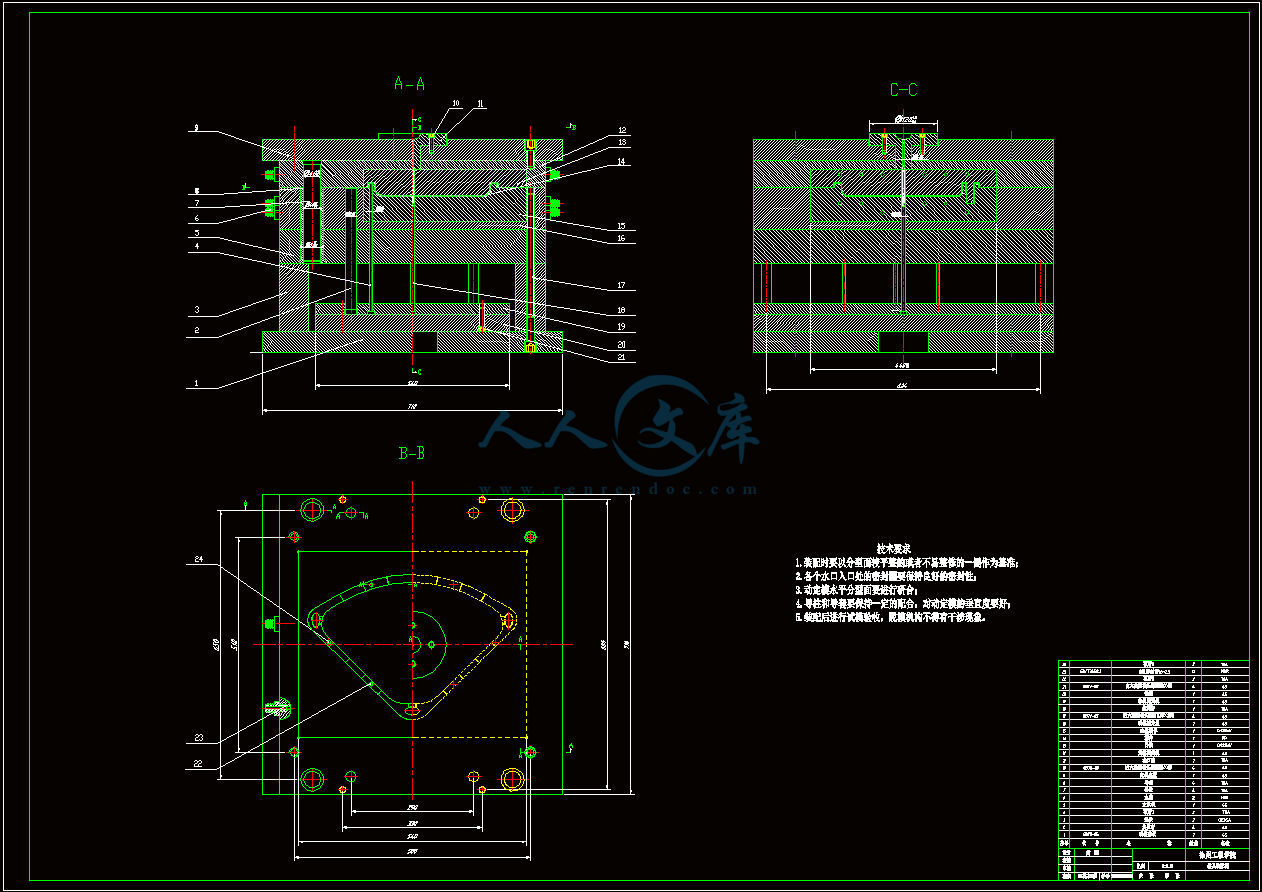
目录
摘要I
AbstractII
1 绪论1
1.1模具工业的地位和发展前景1
1.2课题内容和意义1
2塑件成型工艺性分析3
2.1塑件结构分析3
2.2塑件的工艺性分析3
2.2.1塑件材料的选择3
2.2.2 塑件的壁厚5
2.2.3 塑件的表面质量5
2.2.4 塑件的精度等级6
2.2.5 塑件的脱模斜度6
2.3 PP塑件的注射工艺7
3 分型面的选择9
3.1 分型面位置的确定9
3.2 型腔数目的确定10
4 注射机型号的选择11
4.1 体积质量的计算11
4.2 注射机的选择11
4.3 注射机相关参数的校核12
5 注射过程与模流分析14
5.1 MoldFlow分析软件简介14
5.2 网格划分14
5.3 塑件的最佳浇口位置分析15
5.4 塑件的流动性分析16
5.4.1 气穴和熔接痕16
5.4.2 注射位置处压力17
5.4.3充填时间17
5.4.4 锁模力18
5.5塑件的冷却分析18
5.6 MoldFlow分析总结19
6 浇注系统的设计20
6.1 浇注系统的设计原则20
6.2 主流道的设计和计算20
6.3 浇口的设计21
6.4校核主流道的剪切速率21
7注塑模具成型零件及模具体的设计22
7.1成型零件的结构设计22
7.2成型零件钢材的选用22
7.3成型零件工作尺寸的计算22
7.3.1凹模径向尺寸的计算22
7.3.2凹模深度尺寸的计算23
7.3.3凸模径向尺寸的计算23
7.3.4 凸模高度尺寸的计算23
7.4 合模导向机构的设计23
7.5 脱模机构的设计24
7.5.1脱模力的计算24
7.5.2塑件脱出机构25
7.6 模架的确定26
7.7模架各尺寸的校核26
8冷却系统及排气系统设计27
8.1 冷却系统的设计27
8.1.1 模具温度调节的必要性27
8.1.2 冷却系统的设计原则27
8.1.3 冷却水道的设计27
8.2排气和引气系统的设计29
9模具的装配与调试31
10 基于Mastercam X2的仿真加工33
10.1 Mastercam X2软件简介33
10.2 多用角架搁板凸模加工34
10.2.1 加工坯料及对刀点的确定34
10.2.2规划曲面挖槽粗加工刀具路径34
10.2.3 工件参数设置35
10.2.4 曲面挖槽粗加工实体加工模拟35
10.2.5规划分型面浅平面精加工刀具路径36
10.2.6分型面浅平面精加工实体加工模拟36
10.2.7规划等高外形精加工刀具路径37
10.2.8 曲面等高外形精加工实体加工模拟37
10.2.9 规划3圆鼻刀曲面平行精加工刀具路径38
10.2.10曲面平行精加工实体加工模拟39
10.2.11 规划顶面平行铣削精加工刀具路径39
10.2.12 顶面平行铣削精加工平行铣削实体加工模拟40
10.3生成加工NC代码40
结论42
致谢43
参考文献44
摘要
注射模具是模具工业的重要发展方向,也是衡量一个国家产品制造水平高低的重要标志。模具 CAD/CAE/CAM 技术的应用从根本上改变了传统的产品开发和模具生产方式,大大提高了生产效率、产品品质以及企业自身的竞争力。
本文根据多用角架搁板实物模型进行了模型特征重构,在此基础上基于 PRO/E软件设计出一套合理的注射模具。首先分析了多用角架搁板制件的工艺特点,包括材料性能、结构工艺性、成型特性与条件等,并选择了成型设备。然后介绍了香皂盒注射模的分型面选择、型腔数目及布置形式,重点介绍了浇注系统、成型零件、冷却系统、脱模机构的设计。然后选择模架,并对注射机的工艺参数进行了校核。在此基础上,本文讲诉了如何运用Mastercam软件对多用角架搁板凸模进行仿真加工。
关键词 注射模具;PRO/E;Mastercam;仿真加工
1 绪论
1.1模具工业的地位和发展前景
塑料注射成型所用的模具称为注射成型模,简称注射模。它是实现注射成型工艺的重要工艺装备。塑料模具为模具总量近 40%,而且这个比例还在不断上升。这类模具主要是两类:一类是大型模具,一类是精密模具。大型模具主要是以汽车仪表板,保险杠和家电产品模具为代表的成型模具。精密模具是以集成电路、塑封模具为代表的成型模具。注射模具被欧美等发达国家誉为“磁力工业”。很大部分工业产品依赖注射模具才得以规模生产、快速扩张,由于注射模具对社会生产和国民经济的巨大推动作用和自身的高附加值,世界模具工业,尤其是注射模具工业发展较快,当前全球模具工业的产值已经达到 600 亿~650 亿美元,是机床工业产值的两倍,其中注射模具工业的产值已经达到 240 亿至 260 亿 。
纵观发达国家对模具工业的认识与重视,我们感受到制造理念陈旧则是我国模具工业发展滞后的直接原因。模具技术水平的高低,决定着产品的质量、效益和新产品开发能力,它已成为衡量一个国家制造业水平高低的重要标志。因此,模具是国家重点鼓励与支持发展的技术和产品,现代模具是多学科知识集聚的高新技术产业的一部分,是国民经济的装备产业,其技术、资金与劳动相对密集。目前,我国模具工业的当务之急是加快技术进步,调整产品结构,增加高档模具的比重,质中求效益,提高模具的国产化程度,减少对进口模具的依赖。
现代模具技术的发展,在很大程度上依赖于模具标准化、优质模具材料的研究、先进的设计与制造技术、专用的机床设备,更重要的是生产技术的管理等。21世纪模具行业的基本特征是高度集成化、智能化、柔性化和网络化。追求的目标是提高产品的质量及生产效率,缩短设计及制造周期,降低生产成本,最大限度地提高模具行业的应变能力,满足用户需要。
在科技发展中,人是第一因素,因此我们要特别注重人才的培养,实现产、学、研相结合,培养更多的模具人才,搞好技术创新,提高模具设计制造水平。在制造中积极采用多媒体与虚拟现实技术,逐步走向网络化、智能化环境,实现模具企业的敏捷制造、动态联盟与系统集成。我国模具工业一个完全信息化的、充满着朝气和希望而又实实在在的新时代即将到来。
1.2课题内容和意义
本次设计的课题设计内容主要包括以下几点:
1.进行多用角架搁板塑件的三维造型设计;
2.从塑料品种、塑件形状、尺寸精度、表面粗糙度等方面考虑注塑成型工艺的可行性和经济性,进行塑件成型的工艺性分析;
3.根据塑件的质量和模具型腔数大致确定模具的结构,初步确定注射机型号,了解注射机与模具有关的技术参数;
4.考虑塑件成型位置及分型面选择,模具型腔数,型腔的排列,流道布局及浇口位置设置来进行凸、凹模零件设计;
5.将模具三维模型导入CAM软件中,进行注塑成型模具三维型面的数控仿真加工;
6.由模具三维结构图绘制出二维装配图,依据装配图完成模具主要零件的设计和图样绘制。
本次毕业设计通过对注塑成型模具的设计,可了解常用塑料的性能、塑料制品的设计原则及方法,掌握注射模具常用结构的组成、特点及应用场合,并由此熟悉注射成型工艺及仿真加工的全过程。通过学习现代化的设计制造方法,积极开展CAD/CAM技术在注射模具设计中的推广应用, 从而提高自己进行模具设计的工作能力。